Revolutionary Leap in Superconductivity
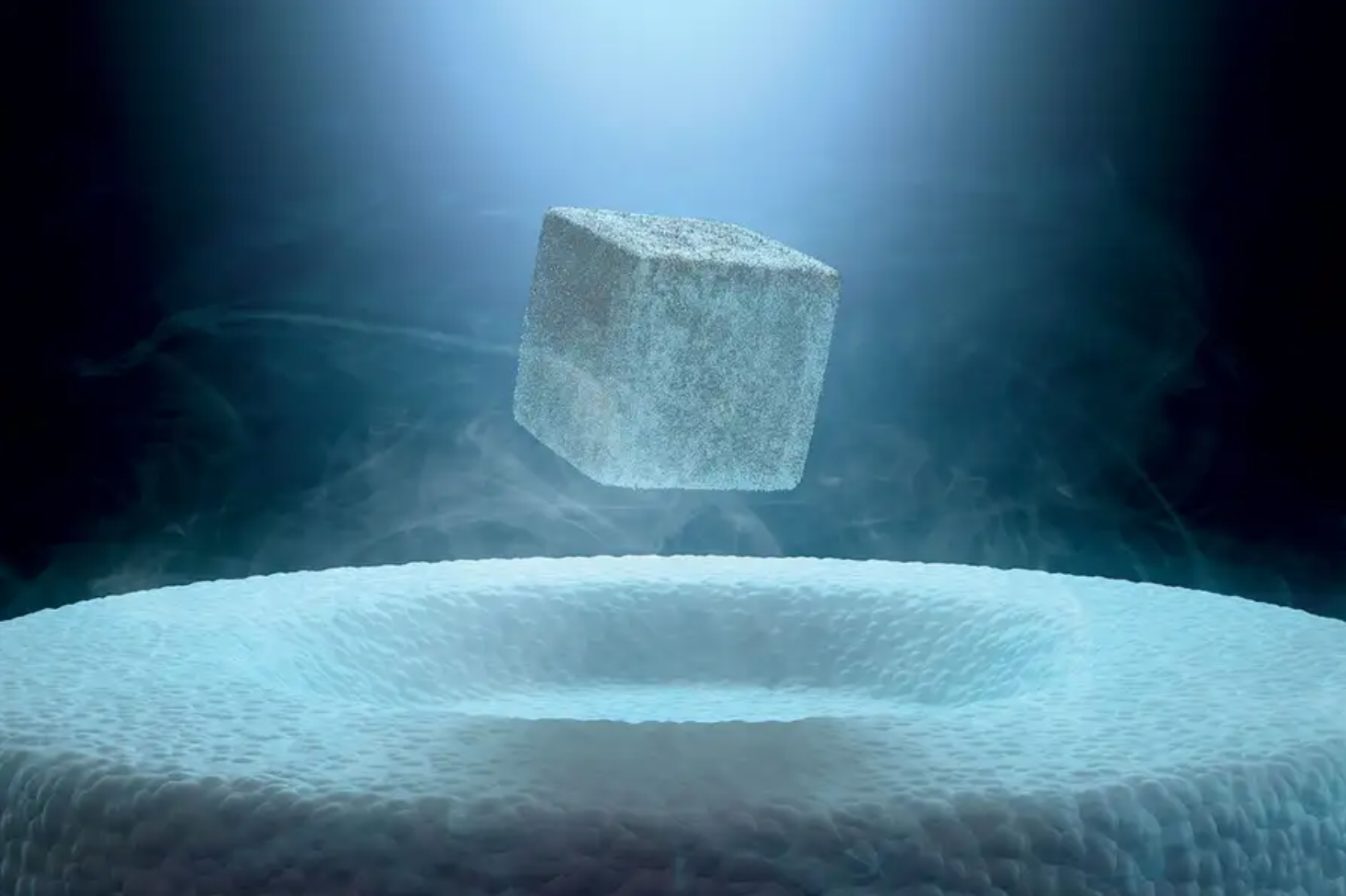
Physicists from the Massachusetts Institute of Technology (MIT) have unveiled a groundbreaking discovery that propels our understanding of how specific superconductors transition into a superconducting state. This revelation promises fresh insights and opportunities for improving existing superconductors and discovering unconventional ones.
Superconductors are materials that, under particular conditions, typically extremely cold ones, transform their structure to unlock a new, superconducting behavior where electrons can flow entirely friction-free. This structural shift is termed a “nematic transition.” It is believed that this transition could be a novel way to instigate materials into a superconducting state, but the exact driver of this transition remained elusive until now.
The team of MIT physicists made this significant discovery by studying iron selenide (FeSe), a two-dimensional material recognized as the highest-temperature iron-based superconductor. Iron selenide switches to a superconducting state at temperatures as high as 70 kelvins (close to -300 degrees Fahrenheit). While this is still ultracold, the transition temperature is higher than that of most superconducting materials.
The concept of “nematicity” originates from the Greek word “nema,” meaning “thread.” In the realm of physics, nematicity is used to describe a coordinated shift that propels a material into a superconducting state. The interactions between electrons cause the material to stretch infinitesimally, akin to microscopic taffy, in a particular direction, allowing electrons to flow freely in that direction. The driving interaction behind this stretching has been a point of intrigue for scientists.
Contrary to previous assumptions, the physicists found that iron selenide’s transition into a superconducting state doesn’t involve a coordinated shift in atoms’ magnetic spin. Instead, it involves a collective shift in atoms’ orbital energy, a subtle yet crucial distinction that opens a new pathway to discovering unconventional superconductors.
“Iron selenide has the least clear story of all these materials. In this case, there’s no magnetic order. So, understanding the origin of nematicity requires looking very carefully at how the electrons arrange themselves around the iron atoms, and what happens as those atoms stretch apart,” says Sanchez, an MIT postdoc and NSF MPS-Ascend Fellow.
In their research, the MIT team worked with ultrathin, millimeter-long samples of iron selenide. They mimicked the structural stretching that occurs during a nematic transition by physically stretching the iron selenide samples. Using ultrabright X-rays, they tracked how the atoms in each sample moved and how each atom’s electrons behaved. Eventually, they observed a definite, coordinated shift in the atoms’ orbitals, signaling a new mechanism of nematicity, and superconductivity.
“What we’ve shown is that there are different underlying physics when it comes to spin versus orbital nematicity, and there’s going to be a continuum of materials that go between the two. Understanding where you are on that landscape will be important in looking for new superconductors,” says Occhialini, an MIT graduate student.
